Integration of Distributed Intelligent Virtual Power Plants with Smart Net-Zero Technologies
Our laboratory has pioneered the development of an innovative concept known as the Intelligent Virtual Power Plant (iVPP), a decentralized smart power system architecture built upon a cloud-based grid infrastructure. This system constitutes a large-scale Intelligent Energy Management System (iEMS), which integrates and coordinates the operational status of various Distributed Energy Resources (DERs) and aggregates power from diverse technological sources.In alignment with Taiwan Power Company’s (Taipower) implementation of ancillary service mechanisms, this research advances the traditional Virtual Power Plant (VPP) concept by incorporating green and carbon reduction elements. The resulting iVPP model is designed to support enterprises in participating in Taipower’s ancillary service markets while simultaneously addressing carbon reduction and energy dispatch for sustainable development.To establish a fully functional iVPP architecture, we are progressively developing a central dispatching platform (iVPPC) and regional iVPPn units across the North (N), Central (M), South (S), and East (E) of Taiwan. These components will be integrated with carbon emission management and energy management data. Through the deployment of AI-based optimization modules, the system will determine optimal dispatch strategies for distributed power resources. The objective is to enable the iVPP to satisfy both corporate economic efficiency and net-zero carbon emission targets, achieving an intelligent, sustainable model of decentralized energy coordination.
IEEE : T.-C. Ou*,H. Tieng, T.-H. Tsai, Y.-Y. Li, M.-H. Hung, and F.-T. Cheng, “Design of greenpower clouds for intelligent virtual power plants,” IEEE Transactions onAutomation Science and Engineering (Accepted), May. 2024. (SCI/EI, ImpactFactor: 6.636, Rank Factor:22/270= Q1,first author)
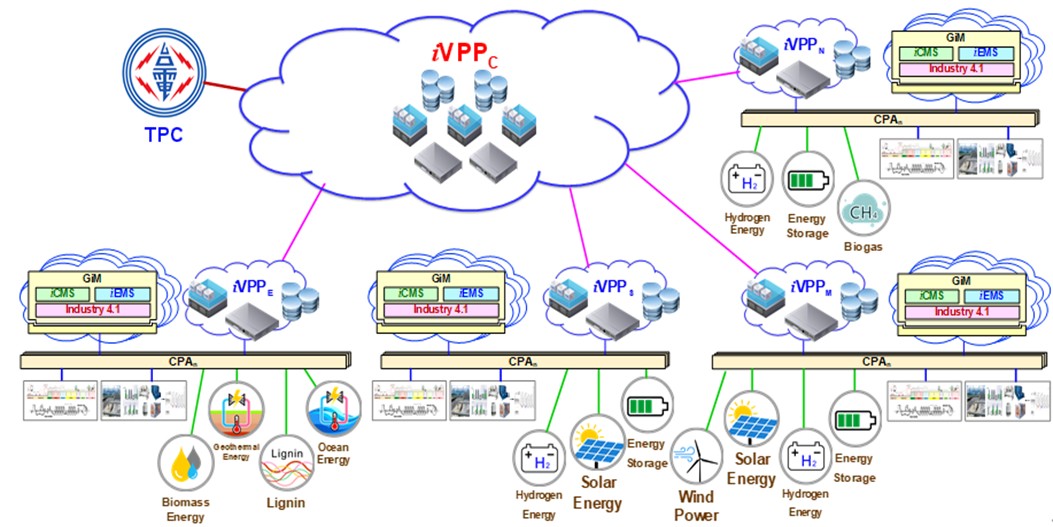
Integrated Smart Net-Zero Solutions for Carbon Emissions and Energy Management, with Intelligent Renewable Energy Control for Carbon Reduction
Our laboratory has developed an innovative hybrid architecture and methodology that integrates carbon emission management with energy management, tailored for applications in Industry 4.0-based green intelligent manufacturing. By utilizing Internet of Things (IoT) devices to collect the necessary big data, and leveraging cloud-based platforms for artificial intelligence-driven forecasting and optimization services, the system provides integrated solutions for both intelligent energy management and intelligent carbon management. This dual-system approach enables simultaneous optimization of smart production processes and smart facility operations, thereby advancing traditional intelligent manufacturing toward green intelligent manufacturing and supporting the transition to green and sustainable energy in pursuit of net-zero emissions.
The newly developed integrated service platform for intelligent energy and carbon management can be applied in conjunction with autonomous smart microgrids and decentralized Intelligent Virtual Power Plants (iVPPs) to achieve optimal control and dispatch of green energy and energy storage systems—including electric vehicles. This system also facilitates the formulation of annual carbon reduction targets while supporting corporate ESG module implementation, thereby accelerating Taiwan’s progress toward net-zero emissions.
IEEE: H. Tieng, T.-C. Ou, T.-H. Tsai, Y. -Y. Li, M.-H. Hung, and F.T. Cheng, “i4.2-GIM: ANovel Green Intelligent Manufacturing Framework for Net Zero”, Early Access (December 2023), IEEE Transactions on Automation Science and Engineering. doi: 10.1109/TASE. 2023.3340149. (SCI/EI, Impact Factor: 6.636, Rank Factor: 22/270=Q1)
Intelligent Energy-Saving and Carbon-Reducing Adjustment (ESCRA)
System with a Set of Carbon-Fixation Biological Negative-EmissionsDevices for Microalgae Cultivation
Surrounded by the ocean on all sides, Taiwan is endowed with abundant marine resources. Among these, the cultivation of microalgae—a form of blue carbon—has recently garnered significant attention. Microalgae possess a carbon sequestration capacity six times greater than that of trees; cultivating one metric ton of algae can absorb up to two metric tons of carbon dioxide, making it one of the most efficient biological pathways for carbon fixation. Microalgae can thrive in high-CO₂ concentration environments, such as those found in flue gas and industrial process emissions from sectors including maritime shipping, petrochemicals, and semiconductors. Once harvested, microalgae can be converted into biofuels, yielding both economic and environmental benefits while supporting industrial carbon capture and carbon cycling, thus effectively reducing direct CO₂ emissions.
Automated microalgae cultivation—referred to as a Carbon Negative Device—involves placing microalgal nutrient solutions into designated containers (e.g., tanks, bags, or ponds) and injecting carbon dioxide to facilitate carbon fixation and biomass growth. Our laboratory has developed an intelligent Energy-Saving and Carbon-Reduction Adjustment (ESCRA) system for carbon-negative biological devices. This system includes microalgae lifecycle identification, a smart carbon credit management platform, and an automated cultivation control mechanism. The cultivation process involves placing the nutrient solution into the selected vessel, followed by the injection of carbon dioxide. After a few days, the cultivation cycle is complete. The harvested algae can be dried for storage or processed into biotechnology products. Through this method, the biological characteristics of microalgae are harnessed to convert CO₂ into organic matter. The resulting biomass can also be refined into bio-oil, making microalgae a highly promising carbon reduction technology and a viable carbon sink solution toward achieving global net-zero targets by 2050.
Globally, interest in large-scale deployment of microalgae cultivation is growing. For instance, the UK-based startup Brilliant Planet has proposed the construction of large-scale microalgae farms capable of capturing billions of tons of CO₂ annually. In Taiwan, several initiatives have already been undertaken. The Industrial Technology Research Institute (ITRI), for example, has implemented systems that use industrial CO₂ emissions for microalgae cultivation, yielding products such as biofuels and high-value biochemicals. National Cheng Kung University (NCKU) has also collaborated with China Steel Corporation to establish a pilot microalgae cultivation facility within the steel plant, utilizing flue gas for CO₂ supply. The system, with a capacity of approximately 2 tons, has been in continuous operation for over two years. The research outcomes are currently being supported and executed under a National Science and Technology Council (NSTC) funded project.

Carbon Capture and Utilization (CCU) , carbon neutrality and negative carbon emission technology
Our laboratory has developed an integrated system architecture for carbon dioxide resource utilization, which encompasses two circular carbon economy models: (1) the development of green energy technologies aimed at achieving zero-carbon electricity, and (2) the advancement of sustainable green petrochemical feedstocks. Key contributions of the laboratory include collaborative participation in the application and execution of Value Creation (VCA) projects, supporting research and analysis in green energy and hydrogen production via bipolar membrane electrolysis, as well as the development and enhancement of Power-to-Gas (P2G) and Gas-to-Power (G2P) technological frameworks.
Further achievements include the evaluation and identification of suitable demonstration sites for carbon neutrality applications, and the construction of a closed-loop, carbon-negative autonomous power generation system by integrating carbon capture with hydrogen energy—thereby forming a net-zero emission green electricity solution. The laboratory has also engaged in the system design of hybrid power generation systems for zero-emission vessels, integrating microturbine technologies, and has proposed the use of synthetic natural gas and partially hydrogenated ammonia as clean fuel alternatives for power generation. These developments aim to assist domestic industries in accelerating their carbon neutrality and net-zero transition pathways. The research outcomes have been formally incorporated into a Value Creation project currently under implementation with support from the National Science and Technology Council (NSTC).
Net-zero building
The building and construction sector accounts for nearly 40% of global carbon emissions, highlighting its substantial potential for carbon reduction. Achieving net-zero emissions by 2050 requires comprehensive research spanning materials, energy efficiency, and carbon mitigation strategies. Our laboratory is engaged in the design of net-zero carbon buildings by collecting and analyzing a wide range of data to identify effective carbon-reduction design methodologies. These include minimizing the use of high-emission construction materials and incorporating carbon-negative materials.
Specifically, the adoption of carbon-negative cement is explored to significantly reduce the embodied carbon of construction materials. In addition, our laboratory has introduced Generative Adversarial Networks (GAN), a form of generative AI, to enhance data-driven intelligent management of green energy and building energy systems. This approach aims to reduce operational carbon emissions through optimized energy usage. Furthermore, carbon management strategies such as microalgae cultivation, biochar application, and afforestation are integrated to offset emissions produced during the construction and operational phases of buildings, thereby accelerating the realization of net-zero building objectives.
Our laboratory has developed a comprehensive design framework for net-zero buildings, integrating both energy management and carbon reduction systems. This framework offers the construction industry an innovative approach to decarbonization and a holistic concept for designing and implementing net-zero systems.

Zhangbin Hydrogen Industry Circulation Park Village Optimization Research
Our laboratory has developed a system architecture for the hydrogen energy industry chain, along with an optimization framework for green hydrogen production within circular industrial park clusters. Hydrogen energy is widely recognized as an indispensable component in the global transition toward net-zero emissions. However, hydrogen-related technologies and the integration of supply chains in Taiwan are still in the early stages of development. Therefore, this research conducts an in-depth investigation into the hydrogen energy supply, transportation, and application segments by reviewing existing data and literature, and benchmarking international technological progress, regulatory frameworks, and standards.
On the supply side, the laboratory has proposed a preliminary blueprint for a hydrogen circular economy cluster that integrates hydrogen production and end-use applications—centered on the Changhua Coastal Industrial Park. Regarding the transportation segment, a comprehensive analysis was conducted on international best practices and emerging technologies in hydrogen transport. On the application side, our laboratory has compiled and analyzed global standards and regulations relevant to hydrogen and fuel cell development, and conducted case studies on international hydrogen-based direct reduced iron (H-DRI) steelmaking projects.
Furthermore, we assess the potential challenges and future research directions across each stage of Taiwan’s hydrogen energy supply chain. These insights provide a valuable reference for the future development and integration of upstream and downstream hydrogen-related technologies within Taiwan’s energy industry. Additionally, we have explored hybrid configurations involving natural gas and hydrogen fuel cells as low-carbon energy carriers to support domestic industries in accelerating their transition toward net-zero carbon emissions.

Novel Green Energy Voltage Controller for the Production of Clean Fuels (Hydrogen, Methane, and Methanol)
Our laboratory has proposed a novel design for a green energy voltage controller (Novel Voltage Controller, NVC), which integrates solar energy with a vanadium redox flow battery (VRB) energy storage system to stabilize voltage output for the production of three distinct types of clean fuels: hydrogen, methane, and methanol. The system utilizes solar energy for hydrogen production and captures carbon dioxide (CO₂) for conversion into methane and methanol.
This approach aligns with the global focus on Carbon Capture and Utilization (CCU), which has become one of the most prominent topics in sustainable development. The present research leverages CCU technologies to develop an innovative green energy voltage control system, which can be further extended to support future advancements in hydrogen energy, carbon neutrality, and negative emission green energy technologies.
SCI : T. C. Ou*, “Design of a Novel VoltageController for Conversion of Carbon Dioxide into Clean Fuels Using theIntegration of a Vanadium Redox Battery with Solar Energy,” Energies vol. 11, no. 3, pp. 524-534,Feb. 2018. doi:10.3390/en11030524. (SCI/EI, Impact Factor: 2.707, Rank Factor:56/103=54%)
Development and installation of a new automatic cleaning system for solar panels
Our laboratory has developed an automated cleaning system specifically designed for solar panels. The system is triggered by either a day-night sensor or a scheduled timer, which activates an Arduino-based controller to execute the cleaning process. The cleaning mechanism includes high-pressure water spray components and squeegees. The squeegee assembly adopts a parallel-staggered configuration, which effectively removes surface contaminants and has been patented.
The system operates in two distinct modes: a cleaning mode for dirt removal and a cooling mode to reduce panel temperature, both of which can be selectively activated based on site-specific operational requirements to enhance energy generation efficiency. Once installed, the system requires no manual operation or relocation during the cleaning process, significantly reducing labor costs. It demonstrates both technical feasibility and market potential, with a hardware cost lower than that of commercial cleaning robots.
A cost-benefit analysis comparing the system with industry-standard cleaning solutions under identical power plant scales revealed that this system reaches breakeven three years earlier and achieves a higher internal rate of return (IRR). These results confirm the system’s economic viability and its suitability for practical deployment across various types of solar power plants.
Wave energy
Our laboratory has developed a wave energy–flywheel integrated system that addresses the intermittent nature of wave power generation through the application of flywheel energy storage. By controlling the energy input and output of the flywheel, the system releases stored energy when wave energy generation falls below grid standards, and stores excess energy when generation exceeds those standards. This enables a stable and regulated power output, enhancing the reliability of wave energy systems.
Furthermore, by utilizing carbon-negative cement in place of conventional cement for structural components, the system achieves a significantly lower carbon footprint. Compared to traditional oscillating water column (OWC) converters, the integration of carbon-negative materials reduces carbon dioxide emissions by up to 121%. The total carbon absorption capacity of a single wave–flywheel integrated system is equivalent to that of 16 Daan Forest Parks, underscoring its potential as both a renewable energy innovation and an effective carbon mitigation solution.
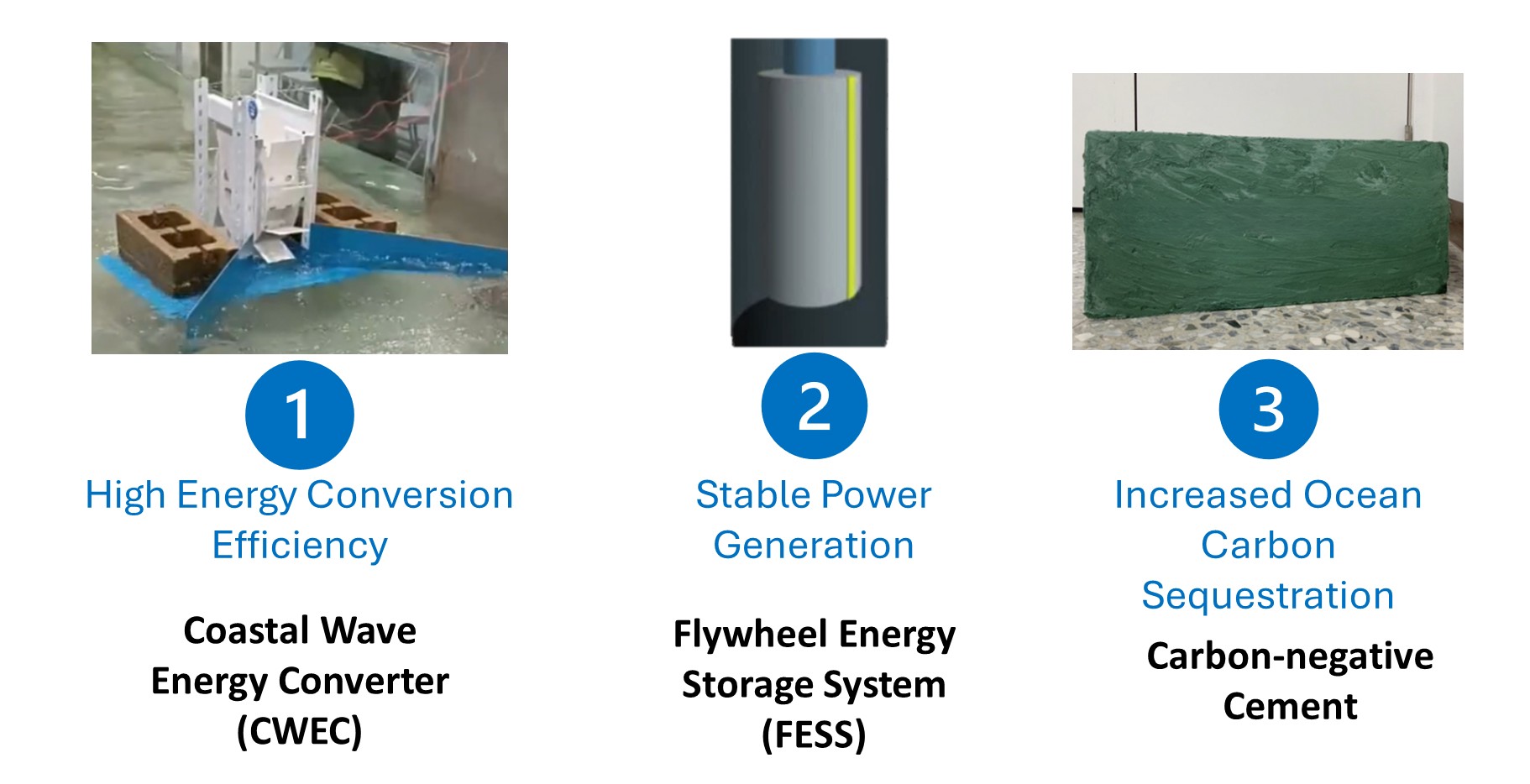
Wind energy
Our laboratory investigates the integration of wind and wave energy systems as an emerging approach to offshore renewable energy utilization. By combining wind power and wave energy generation technologies, this system enables the simultaneous capture of both resources within the same maritime area. Such integration enhances the power generation per unit area and improves the overall stability and efficiency of energy output.
This research examines the structural configuration of hybrid wind–wave energy systems and highlights the synergistic benefits of co-generation. These include more stable power output and enhanced operability and maintainability of offshore wind turbines due to the wave energy converters’ stabilizing effect on sea surface conditions. Additionally, the study explores key challenges and corresponding solutions in the design of hybrid systems, including mechanical integration, control strategies, and environmental resilience.
Cost-benefit analysis and performance assessments were conducted to evaluate the energy yield, construction complexity, developmental feasibility, and long-term potential of such systems. Furthermore, the environmental impact of integrated wind–wave energy systems was analyzed, focusing on noise, vibration, and shadow flicker effects. The results suggest that the integration of wind and wave energy can not only increase renewable energy efficiency but also provide a viable pathway for sustainable offshore power generation.